
Naama Barkai Lab
Department of Molecular Genetics
Weizmann Institute of Science


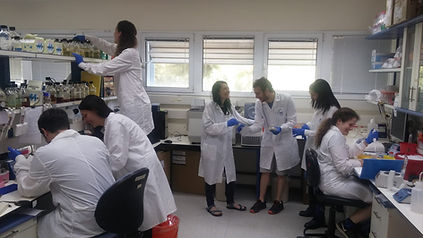
Principles of gene regulation:
The roles of disordered protein regions
Cells have a remarkable capacity to rapidly alter their transcriptional program during development or adaptation to changing conditions. Transcription factors (TFs) are key to this process; they bind to specific gene regulatory regions and induce (or repress) expression upon activation. While we know much about gene expression regulation, fundamental questions remain and lie at the heart of our research:
How do TFs specifically recognize their short binding sites (6-11 bases) within the vast, billion-base pair genome?
How can TFs locate these binding sites rapidly, within minutes of activation?
Theory and experiments demonstrate that the paradigm of a small, structured DNA binding domain in TFs is not sufficient alone for these attributes. Instead, much of the information lies in Intrinsically Disordered Regions (IDRs) which are abundant within TFs. We aim to elucidate the molecular code, or grammar, that enables specific genome-binding selection by IDRs in TFs.
Featured Article
July
2023
The architecture of binding cooperativity between densely bound transcription factors
Offir Lupo, Divya Krishna Kumar, Rotem Livne, Michal Chappleboim, Idan Levy, and Naama Barkai
The binding of transcription factors (TFs) along genomes is restricted to a subset of sites containing their preferred motifs. TF-binding specificity is often attributed to the co-binding of interacting TFs; however, apart from specific examples, this model remains untested. Here, we define dependencies among budding yeast TFs that localize to overlapping promoters by profiling the genome-wide consequences of co-depleting multiple TFs. We describe unidirectional interactions, revealing Msn2 as a central factor allowing TF binding at its target promoters. By contrast, no case of mutual cooperation was observed. Particularly, Msn2 retained binding at its preferred promoters upon co-depletion of fourteen similarly bound TFs. Overall, the consequences of TF co-depletions were moderate, limited to a subset of promoters, and failed to explain the role of regions outside the DNA-binding domain in directing TF-binding preferences. Our results call for re-evaluating the role of cooperative interactions in directing TF-binding preferences.
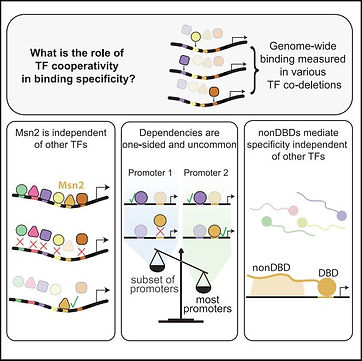
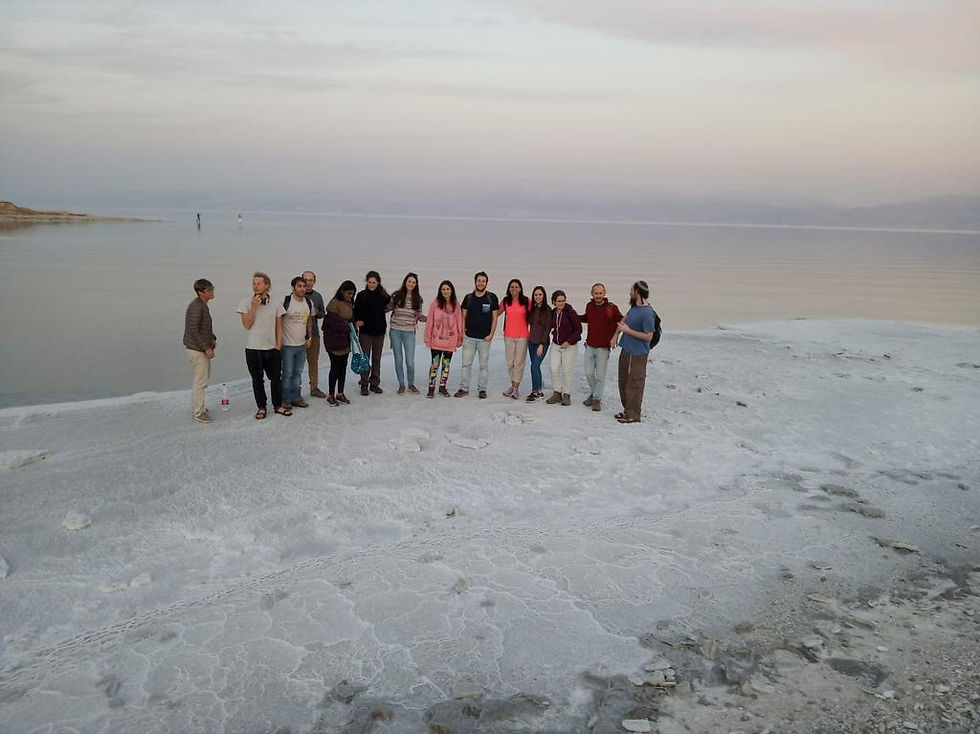

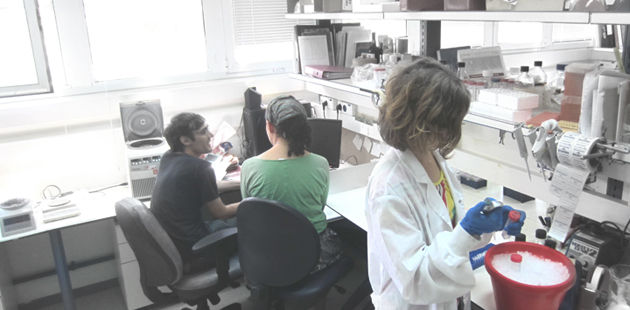
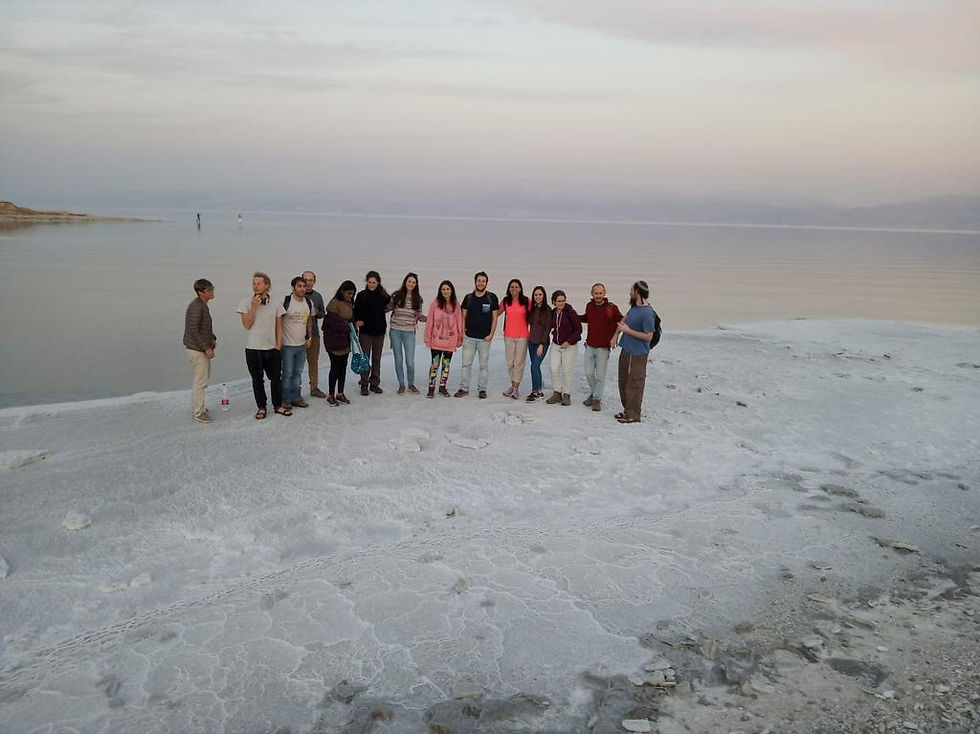